Seamless devices to support our lives
Dr. Philipp Gutruf discusses his biomedical devices that seamlessly integrate with the body to advance scientific knowledge and medical diagnostics.
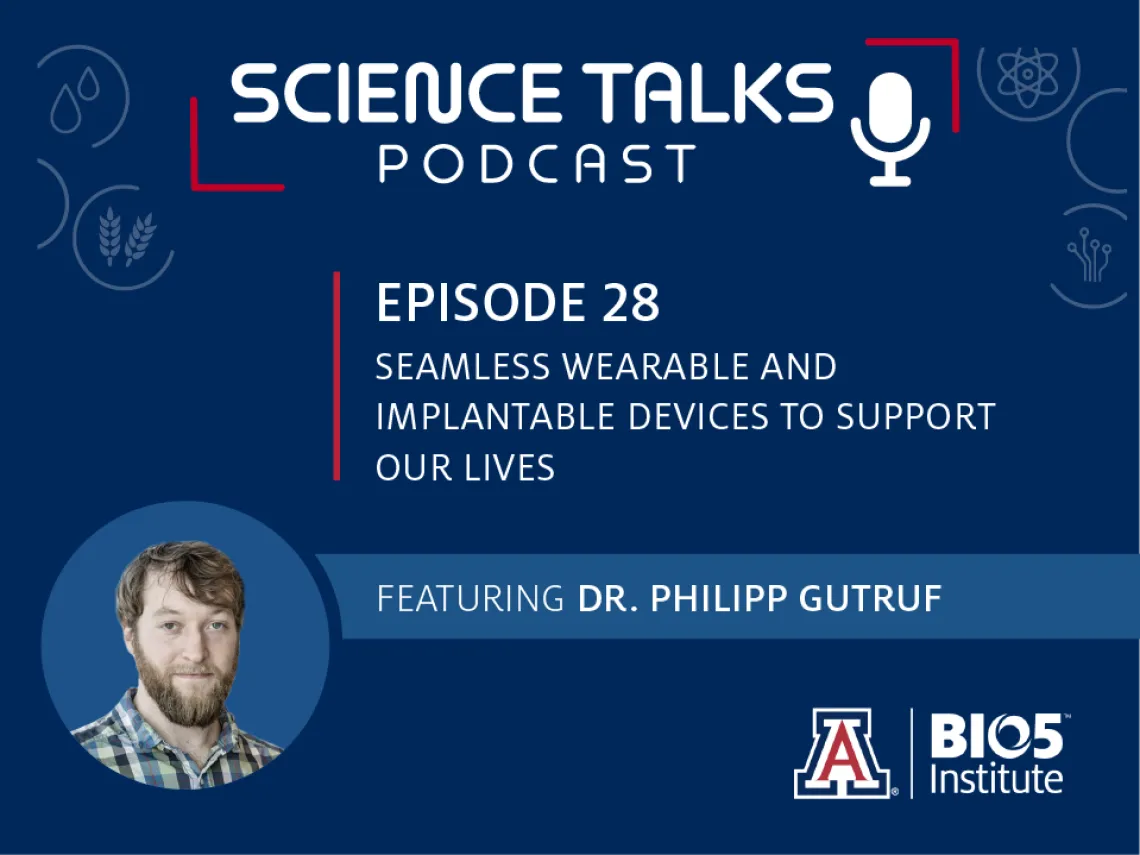
From smart watches to pacemakers, technology is essential in helping us lead happy, healthier lives. Because this tech can be bulky and a little intimidating at times, creating inconspicuous devices that carry a big impact is at the forefront of tomorrow's life-changing innovations. Dr. Philipp Gutruf, assistant professor of biomedical engineering and BIO5 member, specializes in creating new kinds of wearable and implantable devices that seamlessly integrate with living systems to ultimately change the landscapes of health diagnostics and neuroscience.
Your research combines innovations in soft materials, photonics, and electronics to create novel devices. Talk a little bit about how these types of devices are going to help us better understand how the brain works, and how we can enable new treatments or cures for things like chronic pain, depression, and epilepsy?
Let me start by taking a couple of steps back. If you think about contemporary devices that integrate with the human body, for example, this smartwatch on my wrist, it's essentially a brick that straps to you. The same applies also to a pacemaker. It's this large, bulky stainless-steel case, that you get implanted, and the electrodes attach to your heart. The same applies to wearable devices - they get strapped to the body.
One of the fundamental problems is that our body is soft and the mechanics are completely different, in the way that our bodies send information is also very different to our most sophisticated devices. What we are trying to do in my lab is to change the way that bioelectronics or devices that integrate with the body feel and work to create a more intimate contact to the body. That's the baseline of most of our devices.
One of the reasons why a smartwatch is pretty bulky, why a pacemaker needs to be that big, or why brain interfaces have pretty big cables and you need to penetrate your skull to get these devices in, is mainly because the way that we power our devices relies on batteries…and batteries are pretty big. That's not only a problem for biomedical devices, but for cars and planes as well. We are taking these platforms that we try to make as “biology-like” as possible, and we integrate them with means to power them remotely. We use electromagnetic waves that cast power into devices, or wireless power transfer that keeps these devices going 24/7. That's the technological foundation for most of our devices.
Taking a step back, how did you come into this field? How did your training help you to build a foundation, and how do you adapt to technology that changes so rapidly?
I think, in general, the ideas are not necessarily new. If you look into science fiction, the idea or dream is that you just go about your daily life, and everything else happens - we can remotely tell how you're feeling, what your current status of health is, and make diagnostics well in advance. I think that the general dream is not being interrupted with what you're doing daily and not having to worry about healthcare or going to the doctor. The best thing would be if this would all happen automatically. Obviously, technology needs to catch up to this, and we need to find ways in order to interface with the body in a way that allows for the seamless interaction.
A little bit about my background - I come from Germany, and my undergraduate degree was in sensors or sensor system technology. Then proceeded to do a PhD in Australia in electronic materials, so that was my introduction into making devices stretch and bend. I looked into, for example, oxide material that allows us to make stretchable sensors or photonic devices that can flex. The emphasis there was always to enable current technology to be more mechanically compliant to be closer to human body mechanics.
Then I proceeded to do two postdocs. My group at Northwestern pretty much pioneered some of the soft electronic approaches. What I developed were capabilities to interface with the brain and to collect biomarkers from the skin.
Now in my group, we're really trying to emphasize these technologies and bring the unique experience in wireless electronics to this platform to enable very seamless interfaces, whether that be for the central nervous system or for other wearable devices. The goal is that you go about your daily life, and everything else happens.
It's clear your work is very interdisciplinary, which is exactly at the heart of what we're all about at BIO5. Do you find yourself collaborating with people from other disciplines to be able to bring in that outside expertise to help you inform how you make your devices?
Of course, it’s essential.
Biomedical engineering is my home department. All of the possibilities, especially here at the University of Arizona, really help us to do our research. We're just a couple of steps away from the hospital.
Just to give you one example why that's super important - we have this new device class we call osseosurface electronics. These are thin electronic sheets of paper as literally as thick as a piece of paper that allow us to capture a lot of information about bone health. We can grow them on to the bone to form a permanent interface, power them wirelessly, and then extract the data also completely wirelessly.
The initial impulse came from a conversation just after I started at the University of Arizona a couple of years back. Folks from the med school had research projects where they laminated strain gauges onto the bone in order to understand bone health or bone regeneration on the fundamental level. They always would have loved to have this in a form that allows them to take these measurements all the time because that can really inform how we can do therapeutics, for example, and it really informs our basic understanding of bone health in general. There's no means of doing these measurements right now continuously because if you have wires attached, you can imagine there's a large infection risk, so you can do only momentary studies for a relatively short amount of time.
This is really where we can bring our expertise in soft electronics and wireless power transfer to create devices that are very small so that you can then laminate them onto the bone and get that data. This wouldn't be possible if all these people wouldn't be in very close proximity - we can make devices and walk directly over to the hospital and get them tested. Being able to interact with clinician scientists and clinicians to get direct input for the clinical need for these devices, and then be able to react quickly to current ongoing trends, is really important. That's what BIO5 certainly facilitates, and in general, the University of Arizona.
I remember hearing about another project of yours a few years back having to do with songbirds and brain research. Could you tell us a bit more about that?
This is also a project that grew here at the University of Arizona because we have researchers across campus that work with these very rare animal models – songbirds. It's the only animal model that allows us to study how we acquire speech and how the brain deals with learning and also vocalization of speech.
As you can imagine, if you’ve seen songbirds, they’re tiny, so typically the instruments that we have to probe what's going on in the brain are bulky. They don't allow the birds to move around freely, and that is a big problem for songbirds. They don't sing if they're not happy. It's essential for them to practice their song they learned from their parents, and they use it to find their mate, and we can use this cycle in order to understand how the brain works when it comes to speech.
When I arrived here, some people knew about the work that I've done beforehand - being able to create tiny electronics devices that operate without any wires associated with it and that have no real mass associated with them as well. We figured out that we could actually make photonic interfaces to the brain that allow us to modulate certain neuronal groups that they suspected are important for language formation. This project was actually published fairly recently where we could demonstrate for the first time to have these freely flying, untethered animals, where we can modulate the song. We showed if you modulate certain neuronal groups in the brain, you can shift the pitch of that song up or down, depending on the modulation. That is a tool that's now available for the scientific community to really try to understand how the brain acquires language.
You also routinely teach a couple of courses in medical device design and biomedical engineering research. Can you speak to us about your passion for educating future biomedical engineers? What are some of the biggest lessons that you like to pass on to your students?
I really try to diversify the way that I teach biomedical engineering to students. Recently we had the possibility to build a completely new facility in AME that allows us to teach students hands-on skills in biomedical device design. This allows us to do electronics, mechanics, chemistry and biology, all hands on, all in one building. It's really an engineering paradise for biomedical engineers.
I currently teach the BME 310, which is the biomedical device design course. We really emphasize not only designing, but we also build them physically. These students come up with prototypes for devices that they create completely from scratch. We have a competition at the end of the course, and last year's focus was preparing for the next pandemic. They had to come up with devices that allow us to mitigate the devastating impact of these particular events. They came up with really cool devices that manage, for example, stress of first responders or the well-being of people that are isolated. It’s really cool to see the creativity of these fresh young minds.
I also make sure that I have a lot of undergraduate students participate in our research - this is what motivated me.
A quick background story to how I got started in science: I didn't set out to do a PhD. This is something that I walked into. I traveled a lot when I was younger. I was always motivated to find new opportunities in places and new cultures to experience. During my undergraduate research, there was an opening in Australia that allowed me to do research. Beforehand, I hadn't actually done research. I really enjoyed this opportunity - I had this cleanroom available whenever I wanted to, and the supervisor was fairly hands off. They told me, “We would like to have the sensor, and it should be stretchable. Find out a good way to do this.” I really got interested in the process of science, and that allowed me to, for the first time, accelerate my learning as well as my output by completely driving my day by myself and allowing myself to explore.
Before that, I thought I wanted to go into industry, but this was really a turning point for me, so I want to open the same opportunities to undergraduate students here at the University of Arizona.
We have a lot of undergrads participating in research projects at the moment – well over 15 undergraduate students in my lab at this point in time - and they all contribute to projects. We had a couple of students that really used this chance to accelerate their own career. For example, we had an Astronaut Scholarship recipient in my lab that was on several high impact papers. He really was able to build up quite a bit of knowledge in finite element simulation in order to simulate electromagnetics as well as mechanics for these types of devices that we build. There was also a student who is now the first Latinx that got the Oxford Fellows, and she was in my lab for a couple of years.
In general, I really enjoy these success stories by opening up the group, enabling them to do actual research, being able to get on papers to really contribute to the work that we do, to spark that curiosity in the next generation.
Studying can be quite dry and theory heavy sometimes. It's easy to get lost in the coursework and lose the reason why we do this in the first place. If you have these hands-on projects that allow you to really experience and know that you learned this for your transformation, that was always something that motivated me to get through the coursework in order to be a better engineer in the end.
We like to think of our scientists as superheroes. If you were a superhero, what would your superpower be?
I really like to explore opportunities as they come along and leave a little bit of room to act in the moment and not have everything super planned out – it’s actually quite helpful for creativity. I think one of my superpowers is just to act quickly on opportunities that present themselves in the moment.